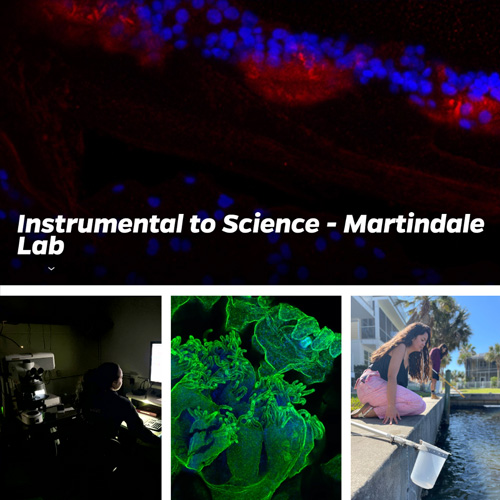
“Everyone knows you need to have the right tool for the job.” — Mark Q. Martindale, UF Whitney Laboratory for Marine Bioscience, UF Explore
Martindale Lab
HIGH TECH: CONFOCAL MICROSCOPY
Understanding basic biological processes requires the ability to magnify images to see cellular (and even subcellular) levels of organization with microscopes. While the fundamental components of microscopes have remained relatively constant over time, technological advancements like computers, laser light sources, and highly sensitive cameras have pushed the boundaries of optical imaging.
Seeing these details of life’s intricacies is a matter of both focusing and filtering light to maximize signal while minimizing background noise. The problem with traditional optical microscopy (e.g. widefield) is that the entire specimen to be observed is illuminated with light, yet only a single plane of that specimen is in focus. In principle this means that the structures that are in-focus are blurred by out-of-focus light.
Confocal microscopy introduces pinholes in the optical path that only allows the light from a single plane of focus to be collected. The drawback is that not as much light is collected, making the image appear dim. Two additional tweaks resolve this issue: the utilization of laser beams that are significantly brighter than traditional light sources and extra-sensitive digital cameras adapted from telescopes that were originally designed to collect light from stars millions of light years away.
Modern confocal microscopes use computers to adjust the focal plane. Researchers can start at the top of any specimen and tell the computer to move the focal plane through the specimen in steps as little as 0.5 microns — less than the width of a bacterial cell. The computer collects in-focus light from each focal plane in the specimen and then reconstructs it in 3D.
These images show a side-by-side comparison of widefield and confocal microscopy. The top panels are two micrographs of the same marine worm. Nuclei are blue. Dividing cells are green. The red fibers depict the worm’s nervous system. As you can see, individual cells and finer structures like nerve fibers are blurred from out-of-focus light in the widefield images (left), whereas confocal microscopy can reveal these finer details (right). The diagrams below the micrographs represent the basic light paths utilized by each microscopy technique to generate their respective images.
Images were taken by Alicia Boyd. Microscopy diagrams were drawn by Brent Foster.
The Martindale lab — and many other biologists at the Whitney Laboratory — utilize confocal microscopes to generate high-res images on a cellular scale. These images are not only full of interesting data — they can also be quite beautiful.
These are images of a developing sea anemone (Nematostella vectensis) showing two proteins (green and red) that become asymmetrically localized during early development. Images taken by former Whitney graduate student Dr. Miguel Salinas-Saavedra.
This is a confocal micrograph of a tentacle of a juvenile Florida Pleurobrachia ctenophore, or comb jelly, stained for filamentous actin (green) and neurons (red). Nuclei are labeled blue. The tentacle runs from upper right to lower left and contains tentacular nerves and “sticky” colloblast cells (small green ‘grapes’ with blue nuclei in the center) that attach to prey items. A small branch of the tentacle can also be seen running vertically on the left side of the image. Under the tentacle is the surface of the animal with its chickenwire-like nerve net (red). Image taken by Dr. David Kainoa Simmons.
This image depicts a close-up view of the bell of an ephyra, or small, immature jellyfish. It has been stained with Dapi (blue), labelling the nucleus of each cell, as well as phalloidin (green), an actin stain labeling the many muscles running around the jelly’s bell. These muscles enable the jellyfish to swim, contracting the bell to push water out, causing the animal to move in the opposite direction via jet propulsion. The “jelly” of the jellyfish, a collagenous gel-like matrix called the mesoglea, serves as a skeleton, supporting the structure and helping the bell expand again after the contraction. The red circles are photosymbiotic algae. In Cassiopea, the upside-down jellyfish, these symbiotic cells are kept in the mesoglea, potentially allowing them to physically move through the animal and better distribute the sugars and other compounds produced by the algae. This contrasts with corals and other symbiotic cnidarians, which just house their symbionts in the epithelial cells lining the stomach. Image taken by PhD student Cody Miner.
LOW TECH: CTENO DIPPERS
Collecting animals from the ocean is not always as easy as it might sound. Many marine organisms are extremely delicate and will fall apart during the collection process. If you drag a plankton net through the water column, gelatinous organisms such a medusa jellyfish, siphonophores, ctenophores or salps will disintegrate before you get a chance to learn anything about them.
Scientists in the Martindale lab collect ctenophores, or comb jellies, from local marinas. These creatures — jelly balls about the size of the palm of your hand — are 99% water and extremely delicate. You can’t even pick a ctenophore up with hands or a net because they will just ooze through and disintegrate. The solution? Attach a beaker that is strong enough to hold water to the end of broom handle or telescopic paint pole. This is not a trivial engineering feat, but Whitney biologists have figured it out using materials from local hardware stores. This piece of equipment is affectionately termed a “cteno-dipper.”
“We used cteno-dippers (i.e., a beaker secured to a broom handle) to carefully collect adult 17 Mnemiopsis leidyi [ctenophores].” Ryan et al. The Genome of the Ctenophore Mnemiopsis leidyi and Its Implications for Cell Type Evolution. Science 342, 1242592 (2013). DOI:10.1126/science.1242592
These excursions to nearby marinas also provide opportunities to engage with local fishermen who are curious about the people peering into the water with a bucket and beaker sticks. Whitney researchers will explain that they’re looking for comb jellies, and the fishermen will usually ask one of two questions: “Can you eat them?” or “Can you use them as bait?”
Whitney scientists don’t eat comb jellies or use them for fish bait. Instead, they are interested in studying the unique biology of ctenophores to understand the molecular mechanisms of regeneration and development. We’re able to heal our scrapes and bruises, but we can’t regrow lost limbs. Comb jellies, on the other hand, can both wound heal and regenerate lost tissues, despite their delicate bodies. One goal of the Martindale lab is to decipher the molecular signatures that distinguish wound healing from regeneration.
Comb jellies also offer unique insight into how early animals evolved molecular pathways that drive development and new tissue types. They have muscles and nerve cells, but no brains. Instead, they develop a “nerve net.” Whitney biologists are trying to understand how this nerve net develops, with the hope that they might learn how the world’s first nervous systems came to be.
BONUS: See how the Martindale lab uses commercially available Rain-X® for science!